Australia's future energy mix in 2100 - a clear case for nuclear energy
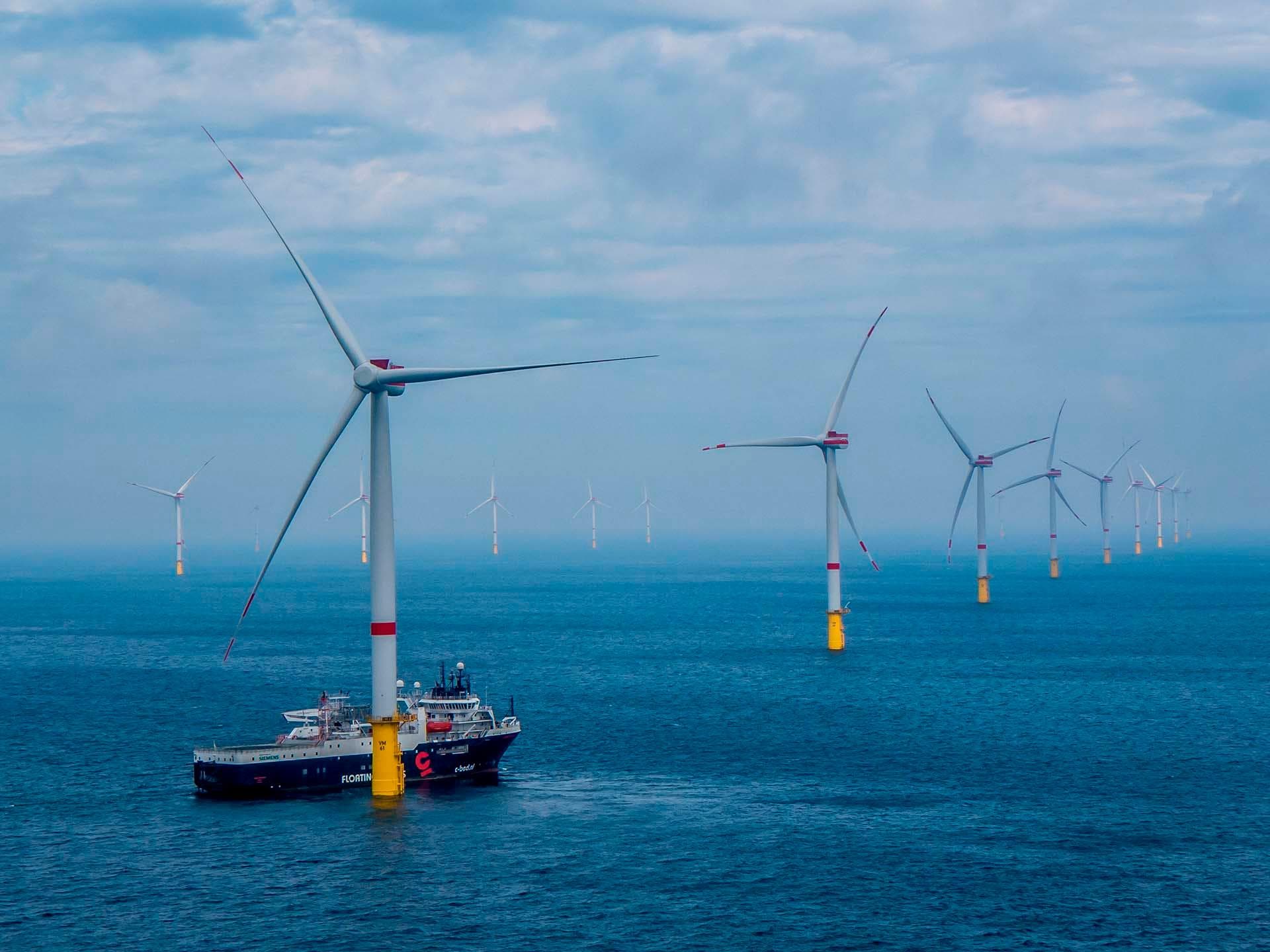
It may seem futile to consider which energy sources Australia will use in such distant futures, but energy production is a massively complex task that requires planning and coordination. Energy is also incredibly political, and to achieve anything meaningful involves shifting public opinion - no easy feat when the subject matter is technical.
Power infrastructure takes between 3 and 15 years to ideate, plan, and then execute. This process relies on the government for legislative needs and policy direction. In 2020, Australia is heavily reliant on fossil fuels for both electricity and other energy needs such as transportation. The rest of the world is in a similar position. By and large, mining fossil fuels and burning them has been the cheapest electricity generation method for a long time.
I got interested in this topic after I read the 2019 Australian Energy Update and realised that Australia still generates almost 80% of our electricity from fossil fuels. After reading various reports from other developed and developing countries, it became evident that Australia's energy generation profile is much more similar to say China or India than it is to France, Norway or Sweden. Policy reform over the last 50 years has been lazy, lacking scientific direction and the cheapest form of power has always won. This probably is heavily influenced by the incredible coal resources we have as a nation, the corporations that profit from this coal and their inevitable influence in parliament.
Anyone that rationally looks at the facts will also understand why we cannot just continue as we have. It is a shame I even have to say this but climate change is very real. Climate change will very quickly uproot the life we live today and create immense problems for generations of 2100. My generation, my kids and their kids will have to live through the effects, so I believe it to be pertinent that we influence those in power to consider us. If our prime minister is bringing coal into parliament, we have an incredibly long way to go. Scott Morrison is not alone in his beliefs. Older generations do not take the science seriously and are often quite apathetic of it.
I approached this article with an open mind; how do we produce the same amount of energy without producing all the CO2 emissions that we currently do? Its a simple open-ended question. After researching in regards to our options, I found that we must consider nuclear energy in addition to heavily investing in renewables. Only this combination is enough. One without the other leaves a reliance on fossil fuels. Renewables and nuclear aren't competing, but rather they are complimentary. Hopefully, in this report, I can explain to you why I believe that is the case.
I didn't intend for the report to be this long, but the more I researched, the more things I felt needed to be included. I could easily write another 50 pages on the matter, but hopefully, the information I included is enough to convince you of a few things:
- Australia is very very far away from the low carbon emissions electricity and transport sector we need. 91% of our energy comes from fossil fuels. We are also improving slowly.
- We are heading towards 3.2C predicted global mean temperature increase. We are already at 1C and we must do whatever we can to release fewer carbon emissions in the atmosphere, and do what we can to take them out. We must also do this swiftly. The effects will make our world much less livable than it is today.
- Solar and wind energy are wonderful sources of electricity, their costs are decreasing year on year and they are low carbon emitters. The drawback of wind and solar is that they suffer from variability issues, viable storage solutions are a long way away and even when they arrive, solar + wind + storage would make for a very flimsy grid. 100% solar and wind is nearly impossible.
- Nuclear energy is a great source of low carbon emissions energy, which can work well in conjunction with solar and wind. Nuclear should provide ~70% of our electricity, and we should have 70% our of demand in installed wind and solar capacity as well. Overflows should be used for carbon capture, hydrogen electrolysis and to create a cheap energy nation.
- Nuclear energy is not nearly as bad as it is made out to be, in fact, it is our best hope. If we do not use nuclear we will need to rely on natural gas. Period. There is no way around it
These are the major sections, which can be easily referred to.
- Australia's 2020/current energy mix: Where do we currently stand? How much energy do we use and where do we get that energy from?
- Why should we change: Considering our current sources, why are they problematic? Basics of climate change
- Energy options: What are our options for changing?
- Solution 1: Solar and wind
- Solution 2: Nuclear
- Conclusion
1. Australia's current energy mix
Thinking about the future is hard. We are seldom accurate. But the best thing we can do is understand where we stand today, and direct ourselves in the right direction. Assessing where Australia stands is vital then.
In this assessment, I want to consider two main areas.
- How much electricity do we consume domestically and;
- How much energy we use for transportation
Together these two account for ~70% of energy consumption, and ~67% of CO2 emissions. Australia is a net exporter of energy, with exports equal to around 66% of production. Of that remaining 34% of production alongside imports, electricity generation and transport are the major consumers. Other contributors include manufacturing, mining and residential non-electricity use (e.g. gas), but these are much more difficult to transform into clean energy-based consumption.
So in assessing a future energy mix, we know that we need to produce enough energy to meet these requirements. We also know that consumption in these areas could be much "more green" by using clean energy and clean transport options - transformations that are the lowest hanging fruit. They also serve as a baseline for identifying an energy mix that produces less carbon emissions.
The categories I will be looking at are thus:
- Domestic electricity consumption: Exclusively the electricity consumed within Australia. This does not include energy exported or wasted energy (e.g. a coal plant is only 30% efficient in converting the energy within coal into electricity, only the 30% counts because that is all we use)
- Domestic transport industry energy consumption: Energy consumed by the domestic transport sector. Again I will only be considering energy output. If all vehicles had output counters in kilowatt-hours that is the number I am interested in.
Focusing on energy output allows us to consider our energy needs without confusion. We know exactly how many kilowatt-hours or petajoules we need from our future energy mix.
These two consumption statistics allow us to establish a rough estimate of the current energy consumption that could be theoretically replaced by electricity. Of course, this is not feasible, and there will always be exceptions, but consider the scenario where everyone in Australia drives a Tesla and all trucks are Tesla trucks. Then the transport sector could be powered by electricity, and the share of energy consumed as electricity in homes does not change.
Domestic Electricity Consumption
After scouring several government websites for quite some time I was unable to find data on electricity consumption by source. What I did find was a lot of statistics regarding electricity generation by source via the Department of Environment and Energy. I will be using this as a rough guide. Most of the statistics I quote are from the Australian Energy Update 2019. These statistics are for the 2017-18 financial year, so they are a little old, but at least they are official and comprehensive. The 2020 report will be released in September, and I can tell you that am I excited.
In 2017-18, total electricity generation was 261 terawatt hours or 940 petajoules. This figure includes all private and public electricity generation in Australia, but sadly also includes electricity used in the production of electricity. This taints the figure slightly, but it also gives us a higher upper bound for a margin of error. Electricity consumed in the production is not very high relative to these numbers.
Electricity generation by renewables has increased steadily the past few years, but the vast majority of our electricity supply still comes from fossil fuels.
Coal remains the majority source for electricity generation at 60% of all electricity generated, with a 3% decrease for the 2017-18 year. Natural gas generation increased 7% in the year now accounting for 21% of electricity generation. Combined, these two serve as the source for more than 80% of electricity consumed and another 2% coming from oil/petroleum fired generation.
Renewables accounted for 17% of electricity generation with a 10% increase in generation from 16-17 to 2017-18. Hydro, wind and solar account for roughly one-third share of the renewables market, and the 2017-18 period saw wind generation increase by 20%, solar by 23% and hydro reduced by 2%. These are very encouraging growth rates. If these rates are maintained, renewable energy could power half the energy market within the next decade and a half. Whether these rates can be maintained are yet to be seen however, and the looming storage problem remains. Each additional percentage added to renewables will cost more and cause more grid integration issues. This growth rate is unlikely to continue. But perhaps a more important question, if these growth rates are not maintained which power source will pick up the slack?
Domestic transport industry energy consumption
This is an interesting statistic. If we are serious about climate change, the transport industry must change. The transport industry accounts 18.9% of emissions, second only to the electricity generation sector with 33.6% of emissions. Another 20% or so comes from other stationary energy uses which could theoretically be replaced by electricity - e.g. gas-based heating. The transport sector is also on an upward trend. For that reason, we need to consider the amount of energy the transport industry consumes, which theoretically could be replaced by electric vehicles.
Refined petroleum (i.e. petrol and diesel) consumption on an energy basis was 2263 petajoules in 2017-18. This is nearly 3 times larger than electricity generation. Diesel consumption alone is larger than electricity consumption.
What we must acknowledge is that not necessarily all energy consumed by the transport sector is consumed efficiently. Sure the sector consumed 2263 petajoules, but what percentage of that was converted to useful power? Wikipedia concludes the energy efficiency of diesel to be approximately 30%, and petrol approximately 20%. Of course, this is a gross oversimplification, but using these figures and the refined petroleum final energy figures, we can estimate the transport sector uses on average of power.
Concluding
So we can see that at present, Australia's energy is HEAVILY dependent on fossil fuels. Out of the ~1700 petajoules of power we consume annually within probably the most renewable sectors, only 9% of our power comes from renewable or clean resources. I say renewable or clean because at present, whilst renewable is a great thing to have and work towards, we need clean energy sources. Often the two are conflated as the same because most renewable sources are clean, however not all clean sources of energy are renewable.
If 91% of the energy we use come from sources that generate significant carbon emissions, how shall we proceed in the future? We must adapt our energy mix to reduce carbon pollution. Australia is far behind in this race, and similarly developed countries produce at least a majority of their electricity from clean and renewable resources.
2. Why should we change our energy mix at all? (and why we must prioritise clean energy)
There is a natural phenomenon that occurs in our atmosphere called the greenhouse effect. Most of us are familiar, but if you are not here goes. And even if you are - always good to jog your memory. Never know when you may need to rebuff a sceptic.
The easiest way to think about the greenhouse effect is that certain gasses (mainly ) in our atmosphere act as sort of a blanket for the earth. They keep heat from escaping.
This phenomenon is one of the major reasons that Earth is habitable and has the ideal conditions to support life. On most planets, since there is no atmosphere or very little, temperature fluctuations can be very large. For example on Mars, with an atmosphere 100 times thinner than Earth the temperature ranges from 20C during the day to -73C at night. This is during summer on the equator.
The one thing to understand with the greenhouse effect is that it can also work too well. If the concentration of those very gasses that act as a blanket becomes too high, the insulation layer is too thick and temperatures on earth start increasing. This is known as the enhanced greenhouse effect, and it is the reason that as our atmospheric concentration of CO2 is rising, the earth is warming. There is a lot of science behind the enhanced greenhouse effect. Below I am going to dive a little deeper. If you aren't interested, I suggest you look at where we stand on climate change.
Scientific Basis of climate change
To understand the The enhanced greenhouse effect first let us think about the regular the greenhouse effect. Earth receives various forms of solar energy from the sun, with electromagnetic radiation arriving as ultraviolet, visible light and near-infrared waves. Some of this energy (26%) is reflected immediately, some directly absorbed by clouds and the atmosphere (19%), most of it (about 55%) is absorbed by the surface of the earth. Since the earth's crust is a much lower temperature than the sun, when this energy is radiated back out, it comes out as purely heat energy (infrared waves) instead of visible light like it arrived.
So in simple terms, the earth is converting some of the visible light into heat energy. The visible light passed through the atmosphere, but the heat energy earth is radiating back is absorbed by the atmosphere. Heat collects in the atmosphere via this process meaning that the atmosphere on the surface is habitable.
The four contributing gases and their contribution to the greenhouse effect are:
- water vapour (36-70%)
- carbon dioxide (9-26%)
- methane (4-9%)
- ozone (3-7%) The enhanced greenhouse effect is an extension of the above-described greenhouse effect. Certain activities such as burning fossil fuels, agriculture and deforestation increase the net carbon dioxide and methane released into the atmosphere, meaning more of the heat that radiates from the earth is trapped than before. The average temperature on earth then increases. (average is important) The GIF below shows this process visually.
If you are interested in further following this increase scientifically, you can look at these pages on NASA, CSIRO or the Australian government or Wikipedia
Where do we stand right now?
It is useful to understand exactly how dire the situation is in a lot of ways. This context gives us a sense of the urgency required in both reducing carbon emissions, and other emissions such as methane and nitrous oxides.
Climate change essentially suffers from the free rider problem. The free-rider problem refers to a situation in which certain entities benefit from a public good (in this case earth itself) but underpays or doesn't pay for that privilege at all. An easy way to think of this is in terms of carbon emissions from burning fossil fuels. Governments and private businesses use the atmosphere as a place to dispose of their carbon emissions. They benefit from the energy produced in burning fossil fuel and releasing the carbon emissions into the atmosphere, but they do not pay for that benefit of releasing by-products. Nuclear has the opposite because we have to pay for waste storage making it more expensive and difficult than if we could just release it into the atmosphere.
In some hypothetical scenario where the release of carbon emissions in the atmosphere was not free, and emissions generators had to pay for the cost of releasing those emissions, we would probably not have a climate problem. The cost of releasing those emissions would be too high and so they would find other ways. Unfortunately, this is not how our world works. Our economic system struggles to accurately price indirect consequences, especially difficult to understand consequences (for some) with a scientific basis. These consequences don't affect us immediately or directly, and we are not evolved to deal with such situations.
With this in mind, let's delve into what the costs of releasing carbon emissions at increasing rates for the last 150 years have been. Firstly let us start with an easy trend. How much has the global average temperature increased in recent years?
This graph, from NASA's Climate Division shows that since pre-industrial times (usually between 1860 and 1880) global mean temperature has increased by about 1C. As you can see, around the 1960s global mean temperature started diverging from total solar irradiance (a proxy for the energy we receive from the sun). It makes sense. Estimates suggest that 85% of anthropogenic (human-induced) greenhouse gasses have been released after WWII, and more than half since 1990.
Plenty of research has been done on the matter in the last 3 decades, starting with United Nations Framework Convention on Climate Change in 1992. Al Gore did his famous documentary in the early 2000s but not much has happened since. Average growth rates in emissions have been steadily increasing, led by increases in the developing world. Here GHG = greenhouse gasses. Source: UN
Countries have slowly adopted greener policies, and probably the most promising thing to have happened is a vast reduction in the cost of solar and wind power.
The most widely accepted work on climate change has been done by the coalition of scientists and policymakers led by the UN, known as the IPCC. The Intergovernmental Panel on Climate Change (IPCC) is an "objective, scientific information relevant to understanding the scientific basis of the risk of human-induced climate change, its natural, political, and economic impacts and risks, and possible response options" (Wikipedia). The IPCC is the gold standard in assessing human-induced climate change. The IPCC is conservative, and only incorporates the latest research which passes the threshold of inarguability.
The IPCC released its Fifth Assessment Report in 2014. If you are interested, it may be worth reading the Synthesis Report which summarises the key observations for policymakers. The report is very clear and definite about human-induced climate change:
- "Human influence on the climate system is clear, and recent anthropogenic emissions of greenhouse gases are the highest in history. Recent climate changes have had widespread impacts on human and natural systems."
- "Warming of the climate system is unequivocal, and since the 1950s, many of the observed changes are unprecedented over decades to millennia. The atmosphere and ocean have warmed, the amounts of snow and ice have diminished, and sea level has risen."
The report links CO2 emissions to global mean surface temperatures "Cumulative emissions of CO2 largely determine global mean surface warming by the late 21st century and beyond. Projections of greenhouse gas emissions vary over a wide range, depending on both socio-economic development and climate policy." The IPCC produces a very useful statistic which shows CO2 ppm as related to mean surface temperature increases:
We can combine this with the latest observational data from NASA:
And a few things become evident:
- The rate of CO2 emissions is not slowing down. We are increasing by about 10ppm every 5 years.
- We are very quickly approaching a definite 1.5C temperature rise. We are likely to get there before 2030.
- The possibility of a 2C increases significantly every year that effective policy is not put in place to mitigate emissions.
Something worthwhile understanding about climate dynamics is that they are widely considered to be non-linear. So every 0.5C increase does not have the same impact. 0-0.5C might have very minor impacts, but 1.5-2C could be much more extreme (as it is widely accepted). An example of why this is the case can be thought of in terms of the positive reinforcement cycles. For example, polar ice caps melting. 0-0.5C might have no discernible impact on the polar ice caps, however moving from 1.5-2C means that the mean temperature increase in the polar areas is large enough to melt ice which kept permafrost submerged. Firstly, the newly uncovered permafrost now releases methane into the atmosphere, further exacerbating the greenhouse effect situation.
There is now also less reflection of heat by white ice and snow but rather absorption by a dark ocean. So the ice caps melting causes more ice caps to melt. These cycles continue and we could get from 2-3C quicker than we got from 1-2C.
The goal of the recent 2015 Paris Agreement was to coordinate on a global scale to keep global mean temperature increases to 1.5C. There is often talk about the 2C cliff, whereby the effects of 2C of temperature increase are significantly worse than any level below. The concerning part is this. Even though the IPCC stated in 2014 (6 years ago) we are likely to exceed the 2C cliff, no serious action has occurred since then.
A recent study included in the 2019 Emissions Gap Report compiled by the UN estimates we have less than a 5% chance of avoiding a 2C increase by 2100, and the likely range is between 2.0-4.9C.
The Emissions Gap Report makes a clear case for just how perilous our situation is. The report indicated that even if all countries meet commitments made under the 2015 Paris Agreement (which they are certainly not on track for) the world is due for 3.2C global temperature rise over pre-industrial levels. The report also shows that emissions need to fall by 7.6% each year over the next decade (globally!) to limit the temperature increase to 1.5C.
The difference between 1.5C and 3.2C is astronomical. A whole book could be written about the impacts of warming on that level. A brief rundown of just how bad it can get can be seen on NASA's climate page
Linking back to Australian energy production
Now that we understand a little more clearly why climate change is happening, what it has to do with carbon emissions and just how bad the consequence will be for us - we can consider this in the context of Australian energy. The reason for such a deep dive on climate change is that I want to stress just how important it is that we as a nation shift how we produce and consume energy. Just in the electricity generation sector and transport sector, we are still 91% dependent on fossil fuels. We need to change, and we need to change very quickly.
I often hear the counter-argument "Australia only produces 1% of emissions globally". To me, this is just not good enough. How can we expect to see any improvement from countries like China and India when Australia with all its wealth and such a small scale cannot achieve this? Australia has been incredibly lucky to have abundant natural resources that led to continuous economic growth and economic prosperity for a VERY long time. The head start in the prosperity race should be utilised.
With coal, oil and gas exports (3.6% of total) added to domestic emissions (1.07%) of the global total, Australia's contribution to the global carbon footprint is nearly 5%. With 0.3% of the population! And we are barely moving in the right direction.
So what are our options?
Note
I am certainly not a pessimist or a negative person. But these are simply realities, and I see them as problems that need to be solved.
We are pumping too much carbon emissions into the atmosphere. This is a simple fact and a problem we need to solve. Therefore we need to do one of two things (preferably both): 1. Reduce the emissions we are pumping into the atmosphere, and let earth's natural systems ingest excess carbon. It is hard but we start with the low hanging fruit such as electricity production and transport 2. Artificially remove carbon emissions from the atmosphere. This is also difficult and the science is not well developed. But it may be necessary. I won't be focusing on this in this piece. I may come back to it later.
3. Energy Options
Context
It will be useful that we have a very clear set of objectives for exactly what it is we want to achieve, and why we need to make policy changes at all.
Firstly, as a global community, we are approaching a difficult period. Climate change threatens most nations, industries and individuals. Humans are accelerating the process through the production of greenhouse gasses. How we power our society to a large degree is responsible for these pollutants. This is the overarching impetus as to why we must change at all.
Australia is a poor performer in this regard. We produce 80% of our electricity from carbon-polluting sources. We are moving very slowly, and some parts are moving in the wrong direction (e.g. gas). We must either accelerate in low emissions production or consider carbon dioxide removal technologies (i.e. geoengineering).
There will be increasing energy demands, and if Australia is serious about climate change we must adopt electric or hydrogen fuel-cell based cars quickly. This means the energy needs for these cars will quickly come back to the grid.
Lastly, Australia has a huge opportunity. This can be a massive industry, employing hundreds of thousands if not millions of people. We have access to sunlight, wind, economic prosperity and even uranium if we need it (more on this to come).
Ultimately, how do we replace the 1550 petajoules of energy we currently get from coal, gas and petroleum with a source that is low in carbon pollution and is feasible at a grid-scale
Criteria for evaluation
As we need to adjust our energy mix going forward, we will need to steer the Australian market towards alternative technologies. How exactly we choose these technologies is up to us, but due to the highly politicised nature of energy, we need a fair and objective system to consider different futures. The main pre-requisite is that these technologies need to release as little carbon emissions into the atmosphere as possible. We also need them now, and we cannot wait for future technologies like fusion reactors. We also need them to be viable economically and integrate well with a grid system that requires energy 24/7
Some of these are harder to compare than others. The common method to assess new energy ventures is using the levelised cost of energy (LCOE). The LCOE is a cost-based measure that allows us to establish the average net present cost of electricity generation over the lifetime of a plant. The formula is complicated and heavily dependent on the underlying assumptions such as cost of capital, fuel costs, fixed and variable operations and maintenance costs, financing costs, and an assumed utilization rate. The biggest limitation of the formula is that it does not allow for any form of insight as to how the energy source matches the demand profile of the grid that it is connected to. This includes:
- Dispatchability - the ability of the power plant to turn on and off as market demands change
- The extent to which the energy source availability matches demand. i.e. peak energy demand is later in evenings, can the energy source provide peak energy at that time?
One thing I found researching for this is that modern electricity is very complex. Estimating costs, impacts and integrating it with grid electricity is one of the miracles of the modern world.
But when we think about it, what are the low carbon energy options?
- Wind
- Solar
- Geothermal
- Hydroelectric
- Nuclear
The developed world has installed most of the geothermal and hydroelectric power stations in optimal locations. Its not an energy source which we can just keep developing. This leaves us with wind, solar and nuclear as sources of energy which generate low emissions.
4. Solar and Wind energy
Most environmentalists and environmental groups will very firmly assert that solar and wind energy sources are all that we require to combat climate change and that all the technologies we need already exist. There are a lot of reasons for this.
Firstly, the cost. GenCost 2019-20, a collaboration between the CSIRO and the Australian Energy Market Operator (AEMO) estimate the LCOE of wind between $53-$66/MWh with a $59.5/MWh midpoint and solar PV between $35-$66 with a $50.5/MWh midpoint. This compares to a new black coal power station estimated to cost between $83-$112/MWh with a $97.5/MWh midpoint, and gas between $67-$114 with a $90.5/MWh midpoint. These figures don't include a carbon tax, a risk premium for the potential of a carbon tax or Carbon capture and storage (CCS) technologies.
The price of new renewable projects has dropped significantly over the past few years and is expected to keep decreasing as more demand enables economies of scale and investment in R&D. Another plus for the renewables sector is that large plants for coal, gas and especially nuclear often require large upfront investment whilst solar and wind can be built incrementally, making the financing options much more attractive to investors. This is very important because ultimately there are only so many funds that go into the energy sector.
I suppose it is fairly obvious to point out that renewables are also low carbon emission sources. If we combine all CO2 equivalent emissions (CO2e) over the life cycle of a fuel source we see the following numbers for each fuel source. This includes mining of materials, disposal of materials and any associated operations emissions. We measure the emissions in grams of CO2e emissions per kilowatt-hour of electricity.
We see here that wind and solar are certainly not perfect, but they are at least an order of magnitude better than the best performing fossil fuel (gas). Recent research in Nature concludes wind, solar and nuclear have many times lower emissions even compared to gas or coal with CCS technology.
Wind power is certainly a winner in this regard, with the only emissions coming from the manufacturing and installation process. Solar (both PV and concentrated) has a significantly higher carbon footprint due to the extensive extraction process for materials required, furnaces during manufacturing and processing of toxic by products. However, all this considered, these power sources still outperform all fossil fuel based technologies by a very large margin (excluding nuclear, but we will get there)
An astute observer might see that these energy sources still generate carbon emissions. There is no source of energy which releases exactly 0 carbon emissions into the atmosphere, however at these lower levels it becomes more feasible to achieve carbon neutrality or even a slight negative balance using carbon capture technologies such as biochar.
Recent private and public investments into renewables have been huge, with renewable capacity added in large quantities worldwide in the last 5 years. The International Renewable Energy Agency (IRENA) concludes there was a 7.4% growth in capacity in 2019 worldwide with almost 54% of that capacity being added in Asia. Wind and solar accounted for 90% of the added capacity.
The trends are also incredibly encouraging. Additional capacity installed is mostly renewable worldwide, and the renewable share is quickly increasing.
Then you have to ask the question; if renewables have low carbon emissions, they are the least expensive energy sources and they are infinite by nature - why do we need anything else?
The issues with solar and wind
Solar and wind-based electricity generation does not come without a few problems. Although in the early 2000s the cost was prohibitive to even try integrating these technologies on a grid level, the last decade has seen several other problems emerge as costs decreased, however they might not disappear as easily as the cost hurdle did.
The biggest problem is the most obvious one. The sun doesn't always shine and the wind doesn't always blow. We humans don't integrate to this fact very well - we require electricity all off the time. Now to be fair, this problem is not as bad as you might think, and on average there is a stable and predictable flow of electricity:
The above chart shows the average daily supply profile in South Australia for the 2018-19 financial year. I am using South Australia as an example since the state is busy transitioning into renewable technologies and had about 50% of the power generated in 2018-19 come from renewable sources. SA is also inside Australia and represents some geographical similarity to the rest of Australia.
Looking at the smoothness of the average supply is encouraging. The demand and supply profile leans into the concept that as long as there is sufficient renewable capacity 95% of demand could be met most of the time, we just need to install excess renewable capacity and the capacity factor becomes less of an issue. And to a certain extent, this is true. This is why we have seen huge increases in figures such as the share of electricity generated by renewable technologies.
However, this problem does not go away that easily - most of the time is just not good enough for modern society. Variability has several relatively serious implications. Firstly, since we cannot rely on renewable technologies 100%, there always needs to be a backup generation source. Another issue is that as we increase the percentage of renewables, the marginal cost of doing so will increase dramatically. Consider moving from 80-90% renewables. This means we need to add another 10% renewables as well as 10% capacity in gas because the existing infrastructure cannot supply that 10% worst comes to worst.
Consider the following chart:
This chart highlights the issue what can happen on a 'non-average' day. In the middle of the day, it was windy and sunny. The excess supply from wind and solar that SA could export incredible amounts of electricity to neighbouring states.
That being said, the inconsistency in supply is a cause for concern. At around 12:15 AM, there was almost no renewable supply, so all the energy needs were met with gas. This highlights the point I made above. When an energy source can go to supplying less than 5% of the installed capacity, there needs to be backup infrastructure. This backup infrastructure a cost, and when the backup infrastructure generates carbon emissions we have even more issues.
The renewable supply also has steep progressions in either direction. Those steep inclines on the wind and solar supplies make it difficult to have them play well with the grid, as the other energy sources need to be able to match those rates of change.
As you might think, this becomes a bigger problem as the percentage of capacity that belongs to renewable energy increases. This is easily the most difficult problem to solve in the process of moving towards a higher percentage of renewable energy. There are other challenges associated with integrating solar and wind power into a grid system including:
- Uncertainty: Predicting output from solar and wind plants is difficult. Seen how accurate the weather is? Same issue here
- Require strong interconnectivity: Since solar and wind is not equally strong in all areas, the entire grid needs to be able to supply electricity to other parts of the grid. This is an economic and environmental cost
We now see that there is an additional cost which is not being factored in - grid compatibility. This is a fairly serious cost in fact. Consider California in the US. California set a 33% renewable energy goal for 2020 - and by recent statistics (34-35%) reached this goal. This meant a fairly aggressive expansion of solar farm and wind capacity over the last decade:
This has unfortunately come at quite a high cost. Between 2011 and 2017 electricity prices rose by five times as much in California compared to the rest of the US. Most of the additional costs can be attributed to excess capacity in the form of gas, nuclear and coal which is required because the renewable capacity cannot be relied upon. And you might think, well this is the cost we need to pay for climate change solutions. And you would certainly be on the right track, but see below for a comparison of France and Germany. Low emissions do not guarantee high costs.
Renewable Energy Storage
These problems have sort of a carbon-friendly (but costly and not feasible yet) solution: energy storage. Energy storage can be used to capture the excess capacity during high generation periods and supply stored power during low generation periods. This comes in a few forms, the most common being battery storage and pumped hydroelectric storage (PHES).
Currently, battery power is considered too expensive to match grid-level storage for longer periods of time. It is a shame because batteries are easy to install, require little space and can ramp up electricity output quickly. Capacity is still an issue, and grid-level energy requirements cannot be supplied with batteries alone. Batteries are more effective as a helper when there is a shortage.
For short periods of time (0-8hrs), battery storage often works out to be less expensive and more feasible per MWh than PHES. An example of this is the Hornsdale Power Reserve in South Australia. Built by Tesla for a cost of US $50M, the power reserve can store 129MWh of electricity capable of discharge at 100MW. This discharge is continuously split up with 70MW (for 10 mins) contracted by the government to provide grid stability (11.7MWh), and 30MW used by owner Neoen over 3 hours (90MWh) to store energy during excess periods and discharge during shortages.
To give you an idea, just South Australia alone requires ~2GW of power at any given time. This means just to power South Australia with a similar battery, even for just 1 hour, would require 20 such plants. This means US $500m extra. For 1 hour! And then you have to ask the question, how many hours is enough? 1? 5? 20?
A Singaporean company called Sun Cable believes it knows the answer. Still in 'proposal' phase, the project intends to build a 10GW solar array and 30GWh of battery storage in the Northern Territory. The installation is to be connected to Singapore by a high voltage direct current cable. 3GW of dispatchable electricity is said to be available at any time. Details are scarce since the project is so young, however Mike Cannon-Bookes' Grok Ventures and Andrew Forrests' Squadron Energy have provided seed funding for the project. Recently the Commonwealth government fast tracked the project to Major Project Status. Some key figures from that release include:
- $22B cost
- 1,500 Australian jobs during construction, 350 ongoing jobs in Australia, as well as indirectly supporting around 12,000 Australian jobs.
- $2 Billion worth of exports annually
I find this development to be incredibly exciting and think it is certainly in the right direction. The price tag seems to be about right - $5B for the 10GW array, $15B for the 30GWh of batteries, $2B for the cable. I am interested to see how the finer details are worked out, considering the largest battery capacity at the moment is 129MWh. 300x jump to 30GWh is big. And although 30GWh seems like a lot, the project is rated for 3GW of dispatchable electricity meaning the batteries would run dry in about 10 hours under peak demand. 10GW of installed capacity also means that on average 3GW would be produced at any given time. For a system rated to produce 3GW at any given time only producing 3GW on average for half of the day with only 10 hours of storage seems like there will defintely be shortfalls. This means the slack will have to be picked up by something else - either gas or coal. I have said it before and will say it again, I am 100% on board with this project, but nuclear should provide the necessary backup - not gas or coal.
This highlights the key issue with batteries at this stage - long term storage is expensive. It is also very hard to say "we are going to install 30 hours worth of storage, and if we are out then we are out" Batteries are material intense, and generally have a lifespan of 15-20 years. All this being said I am very excited to see where this project leads.
PHES becomes much more economically viable for longer duration storage. PHES refers to a power storage method whereby water is pumped to a higher elevation for storage and released to a lower elevation when the electricity is needed:
PHES is a little bit of a harder sell in Australia. Although we have plenty of land for solar and wind power - water is always in short supply. So building artificial damns is difficult. Even if we had unlimited access to water, there is a reasonably large environmental harm in building damns in high rainfall areas. These dams also require very specific geographies.
A report by researchers at the Australian National University in 2017 concluded it would be possible for Australia to have 100% renewable electricity using 90% solar photovoltaics(PV) and wind, with the remaining 10% coming from existing hydroelectric and biomass energy facilities. Using balance techniques such as widespread PV, excess wind capacity and pumped hydro storage and high voltage transmission the LCOE of a 100% renewable Australia could be around $75/MWh. Although the report makes a good case for this scenario, I don't find it to be particularly viable.
The issue with energy storage is that it has not quite been achieved on a national scale. To move to a 100% renewable society using only solar and wind - you would need a LOT of PHES. More than any other country has achieved to date. Then after you have achieved that hurdle, there are lots of other issues. Base load energy is a central pillar to the modern energy market, having no source of baseload energy is dangerous (think hospitals, defence etc).
This brings it into the realm of aspirational as opposed to realistic. There is always significant reserve capacity in the form of coal, gas or nuclear energy plants required even alongside renewables. It makes sense - no head of state wants to bet on the possibility of there not being power, its political suicide.
Variability of supply is a massive issue for solar and wind power. There are other environmental concerns which I won't go into, but they are also worth considering. I raise this point because some consider these technologies to be 'win-win' as if they have no drawbacks. Some of these environmental impacts include:
- long-distance high voltage power lines to and from areas where land is available for wind and solar farms
- large swaths of land dedicated to solar and wind farms - loss of habitat, loss of carbon sink
- bird and animal species killed by solar and wind farms
- large amounts of toxic waste from solar panels
- noise from wind farms
The biggest impact here is probably loss of land/habitat. How big is the impact? Consider this. If everyone consumed as much energy as Australia that would mean we need to dedicate almost 15% of the habitable land on earth to energy production. This is a highly optimistic figure as well.
Reserve Power
Seeing the large issue of variability of supply, what often ends up happening in practice is that solar and wind installations are 'backed up' by alternative energy sources. This comes in the flavour of gas thermal power plants.
Gas power plants can scale up and down production very quickly, allowing for a suitable backup power source for renewables. Gas is cheap, has lower emissions than coal and a fully functioning gas power plant can be built in 2-3 years.
So if we take a pragmatic approach, we can continue to add renewable capacity which will need to come alongside increased gas capacity. The overall carbon footprint will be lower than the current purely gas and coal mixture, and the low cost of gas will balance out the higher cost of integrating renewables to the grid.
There is a third option. Consider Germany and France. Germany has an ambitious goal of using 80% renewable energy by 2050 and has been pursuing capacity upgrades for both wind and solar plants around the country. Germany also wants to phase out all nuclear power by 2022 and coal power by 2038.
In 2019 Germany consumed 42% of their electricity from renewable resources and saw a drop of almost 6.3% in CO2 emissions driven by higher prices for carbon in the European carbon trading scheme. This means 58% of electricity is still coming from carbon emissions generating sources. Retail electricity prices have also increased significantly, with Germany now having the 2nd most expensive electricity prices in all of Europe, driven largely by subsidies for renewable technologies. You might conclude that this is the price we have to pay for low carbon energy, however that is not the case.
France is a perfect example of this. 71.7% of electricity generated in France comes from nuclear reactors, 21.3% from other renewable sources and 7.3% from fossil fuels. In total, that means almost 93% of energy comes from clean sources. This is incredible! If we compare emissions between France and Germany:
It is not even close. This data is a little old, but the statistics have not changed much. A recent study finds Germany still to be producing at least five times as much carbon per kWh of electricity. And maybe you think Germany is an exception but it has been found across the world. If you want to effectively decarbonise your electricity supply, you must use nuclear power.
Consider the following chart which shows which energy sources led to the peak decade of decarbonisation for several countries over the last 50 years.
It is an uncomfortable truth but solar and wind just are not that effective at de-carbonisation on a massive scale. De-carbonisation on a massive scale is what we need.
You may think, low carbon emissions are nice - but how much more does France pay for it? Nothing
In France electricity is almost half as expensive as it is in Germany:
Recently retail electricity prices in France have been increasing as France bows to pressure from Germany to install renewable energy. By 2025 Germany will have spent $580B to make its electricity 2x as expensive and 7-10 times more carbon intense. It is insanity
So you might conclude if nuclear can provide cheap, low carbon emitting electricity that integrates well with the grid (90% efficiency) then why aren't we building nuclear power plants like there is no tomorrow?
5. Nuclear Energy
If we consider the emissions, safety, cost and other issues rationally we would conclude that nuclear energy is our best bet at a low carbon low-cost energy future. I certainly wouldn't say its a flawless energy source and certainly has its issues, but they are small in comparison to the threat of climate change and can mitigate some of the issues of wind and solar.
Many people do not see it this way. Nuclear energy seems to hit a nerve with many, which means there is instantly a negative bias as soon as it is brought up. Unfortunately, nuclear power is losing a social and political battle because the general public is not willing to consider the facts in a climate change world. What often occurs is that nuclear is compared to renewables as if they are competing when in reality this is not the case. Nuclear competes with natural gas and coal.
One of the biggest groups to blame is environmentalists. By and large, they group nuclear with coal and gas and strongly oppose it, lobby against it and constantly critique it in the media. It is hard to fathom why a group so concerned with the well being of the earth would be so against a clean energy source.
Most of the bias against nuclear comes from certain groups and individuals who have manipulated public perception.
Why has nuclear power fallen out of favour?
Since 1938 when Otto Hahn and Fritz Strassman discovered nuclear fission of heavy elements, this process has been difficult to deal with on a psychological basis. After all, some serious tragedies are attached to the concept. WW2, Chernobyl and Fukushima are sore wounds that are very quickly associated indiscriminately to nuclear power, often without much pause to reflect. Nuclear energy often gets caught up in the political landscape as a heated topic that struggles to make headway in terms of legislation. More authoritarian regimes such as China and Russia are embracing the technology. In China, 88 gigawatts of nuclear power is planned to be in construction or operation in 2020 and Russia is building 10 new reactors. However after three reactors melted down at the Tokyo Electric Power Company’s Fukushima Daiichi nuclear plant in 2011, nuclear energy has been on the decline ever since. The OECD's nuclear energy unit estimates new regulations and safety requirements have pushed up equipment costs by 20% since 2011. Japan scrapped plans for 14 new reactors, Germany will phase out all 17 of its reactors by 2022 and France's Emanuel Macron has promised to reduce Frances dependence on nuclear energy to 50% by 2025.
The main issues often raised with nuclear power are as follows:
- safety
- waste storage
- cost and difficulty of financing
- nuclear weapons proliferation
It is fairly easy to dispel the common grievances held in terms of these points, and show that the downsides they have are relatively small and in most cases smaller than other energy sources. I ask you to approach the issue with an open mind.
Why nuclear is safe
The fallout from a nuclear reactor meltdown is very undesirable, and the risk will always exist. The Fukushima disaster after the 2011 tsunami in Japan gives us a relatively good indication of exactly what those risks are - a modern nuclear plant, with modern disaster response procedures, in a densely populated country and the worst-case scenario (a tsunami).
After the Tohoku 9.0 magnitude earthquake and tsunami three reactors at the Fukushima Daiichi Nuclear Power Plant complex suffered level 7 meltdowns. 150,000 people were evacuated (50,000) have still not returned) and there remains an exclusion zone today. The estimated cost of the cleanup is $75 billion, as part of the $202 billion tsunami price tag. Approximately 167 plant workers received radiation doses which elevate their risk of thyroid cancer. Radiation exposure to workers at the plant was projected to result in 2 to 12 deaths. The Japanese government has screened 180,592 people since the incident and not a single case of radiation-induced health deficiencies was found. The most at-risk group, being female infants who live in the area have a 1% higher risk of developing thyroid cancer over their lifetimes. The most intense effects were psychological, due to displacement. Elevated risks of depression, anxiety, alcoholism and psychosomatic symptoms such as radiophobia. A Japanese newspaper, the Yomiuri Shimbun estimates there to be around 1500 deaths related to rushed evacuation by the Japanese government. The tsunami had 20,000 casualties.
This is what happens in the worst-case scenario. The health effects are not ideal but not terrible - if someone has asked me to guess I would've said it was significantly higher because of how negatively the event was portrayed. A study commissioned two years after Fukushima by Friends of the Earth found that “overall the safety risks associated with nuclear power appear to be more in line with lifecycle impacts from renewable energy technologies, and significantly lower than for coal and natural gas per megawatt-hour of supplied energy”.
The most 'significant' cost here would probably be financial - $75B is no small sum. Again, we must consider these financial and human costs in context. Let's consider as an example the deaths attributed to generating 1 terawatt of energy - without any climate change-related deaths. This means costs just from air pollution and accidents. To give some context, 1 terawatt hour of electricity is about enough for 27,000 people for 1 year (based on the EU consumption rates). These rates are based on research published in peer-reviewed literature which can be found here
First thing you will notice is the vast difference between fossil fuels and clean energy technologies. Whether you use the higher end estimate (0.074 deaths per TW) or the lower end estimate (0.01 deaths per TW) nuclear energy is comparable to renewable technologies in terms of deaths. Fossil fuel based energy is incredibly dangerous when considered in this light. This does not even begin to estimate the potential harm, deaths and other issues long term use of fossil fuels will cause via climate change.
Australia is geologically stable and has a very low earthquake or tsunami risk. The main question here is, would we rather have thousands of deaths every year from coal and gas pollution, and the certainty of contributing to climate change which will cause more deaths or do we want to just have the RISK that a nuclear incident could occur? To me this reasoning is flawed.
Something that often is lost in the narrative here is that it is not nuclear vs renewables. It is nuclear vs natural gas and coal. Nuclear and renewables could take Australia to emission-free energy very quickly - they are not in opposition to each other
Why waste storage is not a problem
The nuclear energy industry is probably the only industry that truly takes responsibility for its waste. Radioactivity has made this problem particularly difficult and it is one of the biggest concerns with nuclear energy.
Consider a 1GW nuclear power plant. NSW requires between 8-11 GW of electricity at any given time, so we would need 8-11 such plants. Each year, a 1GW nuclear power plant consumes around 20 tons of fuel and produces 20 tons of waste which is so dense it fits in 2 dry storage casks. Each storage cask about 6m high and 3m wide. You know exactly where it is, it can be stored safely and recycled in the future.
By comparison, a 1GW coal power plant consumes 3 million tons of fuel per year (that is 80 rail cars a day!) and produces 7 million tons of CO2 released into everyone's atmosphere. A 1 GW natural gas power plant would produce about half as much CO2 as a coal-fired plant, still around 3 million tons.
Of course nuclear waste is radioactive, but that is beside the point. As long as it is stored properly in facilities which have been built for the task there aren't any issues. The problem arises because the waste is real and needs to be dealt with. No countries have plans to remove the CO2 waste their coal and gas power plants release into the atmosphere every year, or the toxic elements left behind from solar panels or as a matter of fact any waste that we generate. Humans just store waste on rubbish tips to be dealt with later.
A 2015 Royal Comission into Nuclear energy by the Commonwealth government noted the follwing:
"While plans for geologic repositories are now well advanced in several countries, finding sites for repositories has been problematic. This has been due in large part to a lack of public acceptance. ‘Not in my backyard’ arguments about the siting of repositories have been fuelled by misperceptions of the level of risk involved in radioactive waste management and the operation of repositories. However, some countries, notably Finland and Sweden, have managed this process successfully and with a high degree of public involvement and support. In Australia, industry and Government must do more to inform and reassure the public in relation to these matters.
Transport of radioactive waste is undertaken safely and securely—in sharp contrast to other energy industries. Since 1971, there have been more than 20 000 shipments of spent fuel and HLW over more than 30 million kilometres. There has never been any accident in which a container with highly radioactive material has been breached or leaked. In contrast, in OECD countries over the past 30 years more than 2 000 people have been killed in accidents involving the transport of LPG....
Nuclear power utilities are charged levies to provide funds for the management of the industry’s waste and for the eventual decommissioning of plants. In the US, the Nuclear Waste Fund now amounts to over US$28 billion, while more than US$23 billion has been set aside for decommissioning. These costs are factored into the cost of the electricity generated and the prices paid by consumers.
In contrast, wastes from fossil fuel power are not contained or managed, involve enormous volumes and a range of toxic pollutants that do not decay. Moreover, the cost of the environmental externalities these energy sources create are generally not factored into the price of the electricity produced."
If you take the problem of climate change seriously, we can build a facility capable of handling the next 10 decades worth of waste very quickly. It is also naive to think that humans won't figure out how to deal with the issue. At best the waste will be stored for 100-200 years before we find a new useful purpose for it.
This, of course, comes at a cost, but this is included in the LCOE of nuclear energy which I will discuss next.
The main point is that the fears surrounding nuclear waste are irrational. The waste is difficult to deal with, but all waste is difficult to deal with. Climate change will destroy humanity long before nuclear waste becomes a problem.
Nuclear is expensive. But thats because we want it to be
The most common phrase I have read whilst researching nuclear energy is that "Nuclear power stations are extremely expensive to build. Nuclear cannot compete on a cost basis with wind and solar, which are the cheapest forms of new generation."
This statement is almost entirely correct. What we must always keep at the forefront of our minds is that nuclear energy is NOT COMPETING WITH SOLAR AND WIND. Nuclear energy is competing with coal and gas. I don't know why this is so hard to understand for many people. Its as if the fossil fuel industry has played a brilliant game of turning allies against each other.
As we move to a society whereby we produce low carbon emissions energy, we need a stable, consistent and well understood underlying supply. NUCLEAR! Wind and solar have significant variability issues which mean gas power stations are built to back them up. We want nuclear power stations to back up our renewables, NOT GAS. The narrative needs to change.
If we actually consider the cost of nuclear, we find is a hard question to answer. This is because the country where it is built, the reactor choice size and experience of the firms building it all matter. Regulations often change as reactors are being built, causing cost overruns and delays.
The short answer is that nuclear is quite expensive. Until we develop scale and expertise (e.g. China where the cost of nuclear is comparable to coal) nuclear will be expensive.
When discussing solar and wind I referred to this diagram which shows the predicted cost for a small modular reactor (SMR) in Australia compiled by the CSIRO and AEMO. This report estimates currently an SMR constructed in Australia would generate electricity at a cost between $254-$333 per MWh. This is definitely expensive, compared to the LCOE of wind between $53-$66/MWh with a $59.5/MWh midpoint and solar PV between $35-$66 with a $50.5/MWh midpoint.
Another perspective - In 2018 Lazard released a report comparing the LCOE of different energy sources.
As expected, we see utility-scale wind and solar is by far the least expensive per MWh. We see that nuclear is certainly on the more expensive end of the spectrum costing between $112-$189 per MWh of nuclear energy. The gold diamonds represent the marginal cost of operation after a facility has been fully depreciated. This cost analysis was done on average, irrelevant of country. Of course in Australia, a country which does not have any nuclear facilities the cost would be larger to begin with.
These reports rely heavily on the LCOE calculation which is heavily biased towards wind and energy projects. Installing 1000 MW of nuclear and 1000MW of solar or wind is not the same, and this is not accounted for. Nuclear has a 90% capacity factor meaning on average the 1000 MW of nuclear will output 900MW. The 10% of downtime can be scheduled and is determined by humans Solar and wind vary between 20-30% capacity factor, so you need to install 3000-4000MW of solar and wind to get the same average output. That already triples the cost of wind and solar. Next, solar and wind are much closer to theoretical cost thresholds, and we are unlikely to see a much bigger reduction in costs, whereas the report estimates nuclear will drop to $124/MWh by 2040. Once a reactor design is proven, it is mass-produced and costs decrease dramatically.
Grid integration costs of solar and wind have also not been considered. Long power lines, storage and backup gas power plants need to be built. Even then, we would still produce more carbon emissions using solar and wind. The LCOE calculations so often used to tout the cheapness of solar and wind also have a massive flaw in the discount rate. When using the formula we need to select an appropriate discount rate to use - which represents the opportunity cost of future returns. Because the payback period for nuclear is so much longer, it is incredibly sensitive to this discount rate. Both the CSIRO/AEMO report and the Lazard report don't even include the discount rates used!
Then, we have not even discussed the learning gained from the investment. If nuclear energy received even a third of the investment/subsidies that solar and wind do the technical innovation would reduce costs dramatically.
When we consider these sources of power a bit more fairly we see that nuclear is only seen as expensive because solar and wind benefit immensely from the flawed LCOE calculations.
That being said, one of the major issues is that nuclear power plants have high upfront costs. The French nuclear plant operator Areva estimates that almost 70% of the cost of nuclear energy come from the construction process and capital servicing costs. Capital servicing costs are especially high since a nuclear plant takes some time to construct, at a high price and then takes a while to pay back the debt. This video explains it well if you are interested. This makes the project very risky, and without government subsidies costs and risks are often to high for private market investors.
Australia is the only G20 country where nuclear power is banned by federal law. Developing a nuclear power program here will probably require bipartisan support.
Companies like Bill Gates' Terrapower and Rolls-Royce are investing in what is known as Small Modular Reactor (SMR) technologies. These reactors are much smaller than traditional reactors with lower costs and less risk. These technologies are just not quite there yet though, and need government investment.
The bottom line is that at the moment, yes nuclear is expensive. However, we must invest and pay this cost in the short term, and as adoption increases, we will reap the rewards. Nuclear becomes cheap over time with expertise and infrastructure.
This short term cost is the climate change levy. Nuclear can serve as the central pillar of a modern energy strategy, backing up renewables like wind and solar. These technologies are not in competition.
Nuclear proliferation
This is a weak argument against nuclear power. Nuclear power has done more for nuclear weapons removal than anything else. Megatons to Megawatts is the name of the joint US-Russia program to turn warheads into nuclear fuel and began in 1994 with the intention of converting 20,000 nuclear warheads into fuel.
Nuclear weapons programs are often developed completely independently of nuclear energy programs. Not once in history has there been an energy-to-weapons sequence. Of the 31 nations with nuclear energy programs, only 7 have nuclear weapons programs and in all cases, the nuclear weapons came first.
I won't say much more on the topic because I truly believe it is just fear-mongering.
6. Conclusion and policy direction
Now that we understand a little bit more about how nuclear energy fits into the scheme of things it is worthwhile drawing out a few conclusions and concrete recommendations. The key point to remember here is that I am not against solar and wind. I am an advocate, but in realistic terms we need to reduce our carbon footprint dramatically and we need nuclear to do so.
Recommendation 1 - change Australia's energy mix towards low carbon sources
I have laid out a fairly clear argument as to why we need to change. Our current generation methods produce large amounts of CO2 as a waste product, which is leading to the enhanced greenhouse effect. A big step we can take is to move our grid (and eventually transportation) to the generation of energy without this by-product. We will probably need to remove some of this waste from the atmosphere as well. Our options are wind, solar and nuclear. WE must make use of all 3. We need to diversify our grid with low carbon electricity sources, and although no source is perfect, if we have a careful balance between the three we can achieve stability, low prices and low carbon emissions
Recommendation 2 - make nuclear legal
Nuclear power generation does not necessarily need to be endorsed by the government, but it certainly shouldn't be illegal. This is an incredibly old fashioned law introduced in a very different context. From here we can actually consider the technology on its merits, instead of throwing our hands in the air and saying "it is banned by federal law anyway". We export almost 30% of the global uranium supply but it is illegal to generate electricity? Does not make sense to me.
Recommendation 3 - nuclear for base load
As we have seen solar and wind power still has some serious issues with variability and grid integration. By themselves, they simply cannot power a modern grid. Storage solutions are not well developed and so the trend is that gas stations are built to back up the renewables. Gas still produces carbon emissions on a large scale.
We must move to have nuclear energy serve as our baseload energy generation source. We could adopt a model similar to France, where ~70% of our energy comes from nuclear power, and the remaining 30% split between hydro, wind, solar and biomass. Excess energy can be used for other low carbon endeavours such as hydrogen electrolysis (for transport) or carbon capture technologies.
Recommendation 4 - aim for 150%
We must aim to generate 150% of our electricity needs. This will become vital as there is ongoing electrification of vehicles and carbon capture and storage processes become more important. Excess electricity does not necessarily need to be stored but instead can be freely available for private business.
References and further reading
I was originally keeping track of all the websites and sources I used but the list pretty quickly grew out of proportion. Here are some of the sources I used.
Calculation of land required for wind and solar
Consider how energy consumption grows with time. In Australia we use ~1700 petajoules of energy, and we have a population of around 25M. Thats roughly
This is approximately
If every country in the world becomes as developed and energy hungry as Australia, we would need about:
per year
Consider a world with 50/50 wind and solar. This means we would need:
from both energy sources. This means at any given moment we need:
kilowatts or watts generated at every moment by our 50% solar and our 50% wind.
Research indicates the following about wind and solar power density: "The power density of solar and wind power remain surprisingly uncertain: estimates of realizable generation rates per unit area for wind and solar power span
respectively.". Even if we use the high end estimate for both, the amount of land this would need is astronomical.
The wind 50% would need about
The solar 50% would need about
The earth has about 130 million of habitable land not covered by glaciers, so the combined land use of for energy production is around 15% of land available. Note here, wind uses almost 99% of the land (of the shared total). Solar is much much more efficient.
That is an incredible amount of land. That is an area about three times the size of Australia. And of course plenty of earth is too mountainous, cold or isolated for wind and solar farms